Flow Batteries: The Next Big Leap for Grid-Scale Energy Storage?
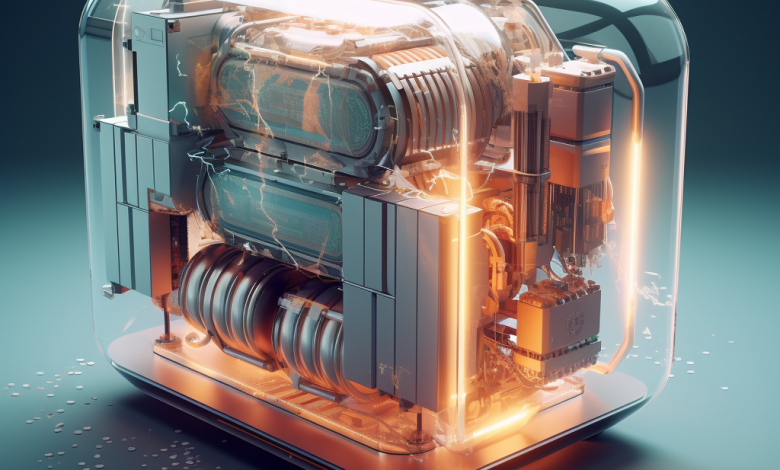
In a world grappling with the growing need for sustainable energy solutions, the role of efficient and scalable energy storage systems has never been more crucial. As the energy sector shifts away from fossil fuel dependence toward renewable resources like solar and wind, a new set of challenges has emerged. One of the most pressing issues is how to store the intermittent energy produced by these renewable sources for later use.
Enter flow batteries— a class of rechargeable batteries that have shown immense promise in providing a balanced and efficient energy storage solution. However, despite their potential, traditional flow battery designs have grappled with issues such as cost-efficiency, scalability, and material limitations.
But a new wave of innovations in flow battery technology is looking to overturn these limitations. By introducing groundbreaking materials and ingenious designs, researchers and engineers are creating systems optimized for grid-scale applications. These advanced batteries could play a pivotal role in stabilizing energy grids, making renewable energy more viable and accessible than ever before.
In this article, we’ll delve into the intricacies of flow battery technology, discuss the limitations of current systems, explore the latest innovations, and examine their relevance and potential for grid-scale applications. Through real-world case studies and market analysis, we aim to present a comprehensive overview of this exciting technology, offering insights into its future trajectory.
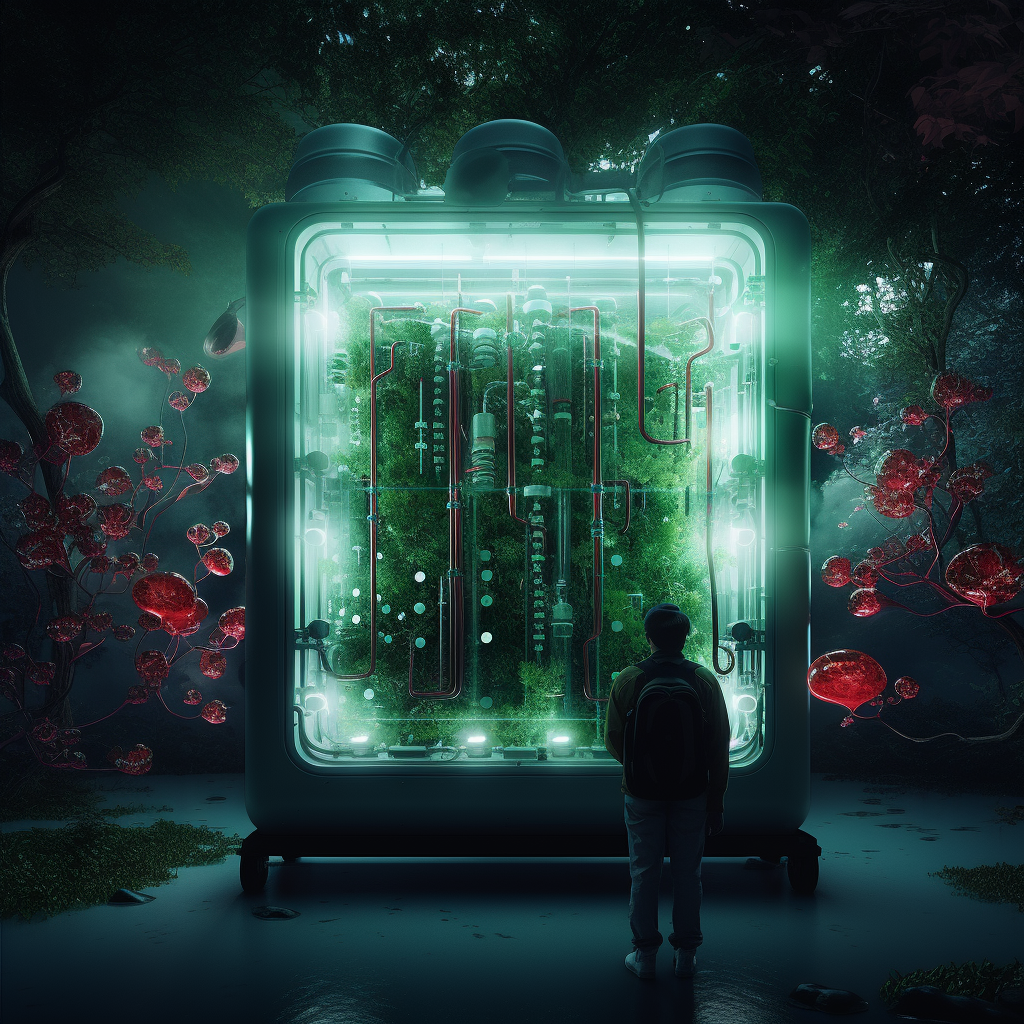
What is a Flow Battery?
Basic Principle
At its core, a flow battery is a type of rechargeable battery where energy is stored in liquid electrolytes, as opposed to the solid materials used in conventional batteries like lithium-ion. The electrolytes are stored in external tanks and pumped through an electrochemical cell during operation. This fundamental architecture allows for some unique advantages, such as decoupling the battery’s power and energy capacity, thereby making it possible to optimize each parameter independently.
Components
A standard flow battery system comprises a few key components:
- Electrochemical Cell: The heart of the battery, where the electrochemical reactions take place.
- Electrolyte Tanks: External storage containers that house the liquid electrolytes.
- Pump System: Facilitates the flow of electrolytes between the tanks and the electrochemical cell.
- Control System: Manages the overall operation, including flow rates and charge/discharge cycles.
Traditional Uses
Traditionally, flow batteries have been used in various applications, from backup power systems to load-leveling for renewable energy installations. Their unique architecture allows for flexible scaling and long-duration energy storage, making them suitable for a wide range of use-cases. However, their adoption has been somewhat limited due to various challenges, primarily related to efficiency and cost.
Limitations of Current Flow Battery Systems
Efficiency
One of the significant barriers to the widespread adoption of flow batteries in grid-scale applications is efficiency. Traditional flow batteries often suffer from lower round-trip efficiency compared to solid-state batteries like lithium-ion. This is primarily due to resistive losses and the energy required to pump the electrolytes between the tanks and the electrochemical cells. These inefficiencies can add up, leading to a less optimal energy storage solution, especially for applications that require rapid charge and discharge cycles.
Cost
Flow batteries come with a unique set of cost challenges. While the raw materials used in some flow batteries can be less expensive than those in lithium-ion batteries, the overall system costs are often higher. This is due to the complexity of the architecture, which includes pumps, electrolyte tanks, and a control system, each contributing to the total cost. The high initial investment required for these systems has been a significant factor limiting their large-scale commercial adoption.
Scalability
While flow batteries offer excellent scalability in theory—thanks to the external storage tanks that can be sized independently of the power-producing components—practical implementation often reveals limitations. These may arise from issues like the increased complexity of larger pumping systems or the logistical challenges of storing and managing large volumes of electrolyte liquids.
Innovations in Flow Battery Design
Material Advancements
The key to unlocking the full potential of flow batteries for grid-scale applications may lie in material science. Traditional flow batteries often use vanadium or zinc-bromine as electrolytes. While these materials are efficient to an extent, they come with their own sets of challenges such as cost and availability. Emerging research is focusing on organic and non-toxic electrolytes that promise higher efficiency and are environmentally benign. Innovations like these not only address the efficiency problem but also make the technology more sustainable and cost-effective in the long run.
Technological Upgrades
Another promising direction is the incorporation of advanced control systems that optimize flow rates, charge/discharge cycles, and overall system efficiency. Utilizing machine learning algorithms to predict optimal operating conditions or Internet of Things (IoT) devices for real-time monitoring are some examples. These improvements aim to reduce the operational complexities and energy losses, thereby increasing the round-trip efficiency of the system.
Importance for Grid-Scale Applications
Energy Storage
The most immediate application for advanced flow batteries is in the field of energy storage. As countries ramp up their renewable energy production, the issue of intermittent power generation becomes increasingly relevant. Solar power is only available during daylight hours, and wind power is unpredictable. Advanced flow batteries, with their scalable and long-duration energy storage capabilities, can store excess energy during peak production times and release it when the demand surges, thereby stabilizing the energy grid.
Balancing Renewable Energy Sources
Flow batteries can act as a buffer between renewable energy production and demand, enabling a more efficient and reliable grid. By storing excess renewable energy, these batteries help balance the grid, ensuring that the energy production and consumption curves align more closely. This capability is critical for reducing the reliance on fossil fuel-based “peaker plants,” which are often activated during periods of high demand, contributing to greenhouse gas emissions.
Market Opportunities
As the world increasingly moves towards greener alternatives, the market for sustainable energy storage solutions is burgeoning. According to a report by Grand View Research, the global flow battery market size was valued at USD 187.0 million in 2019 and is expected to grow at a compound annual growth rate (CAGR) of 30.7% from 2020 to 2027. The advent of advanced flow battery technologies could catalyze this market growth, providing ample commercial opportunities for manufacturers, utilities, and end-users alike.
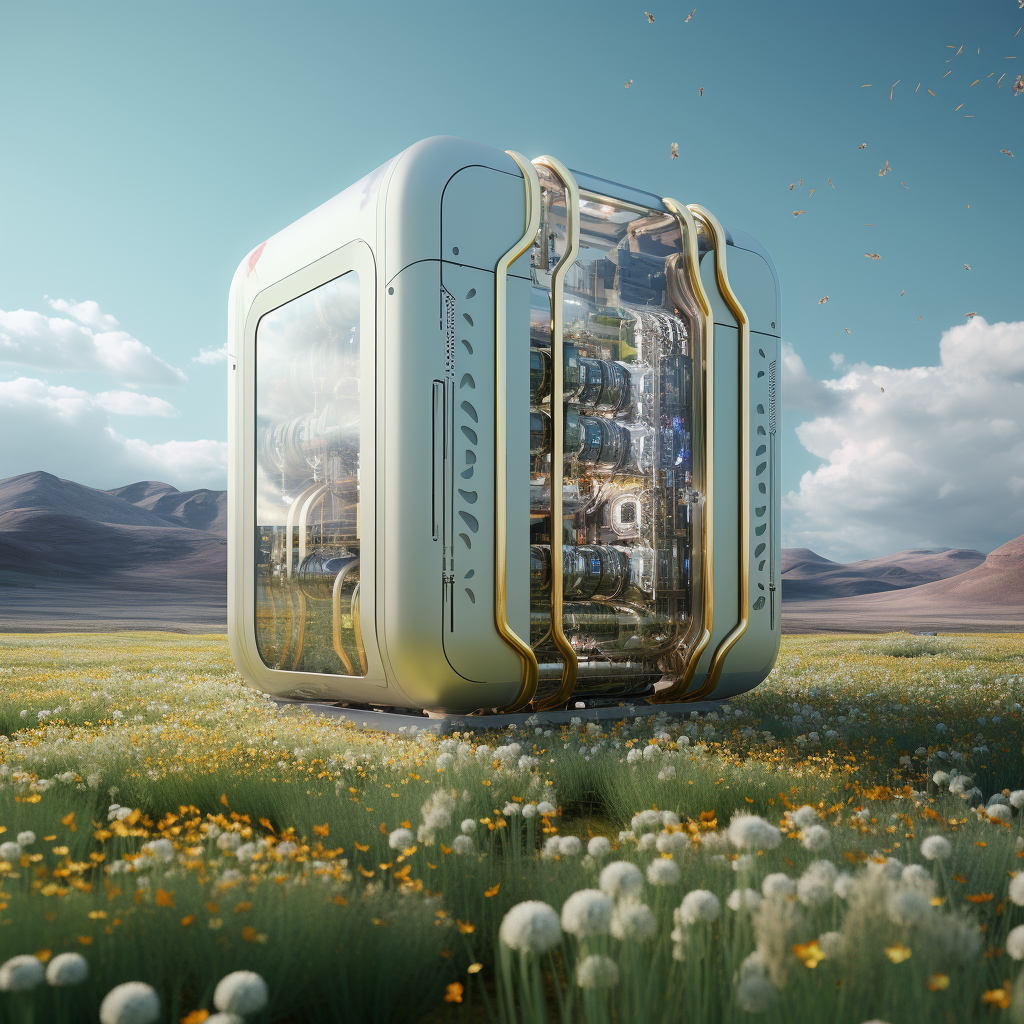
Real-world Case Studies
Pilot Projects
Several pilot projects around the world are currently testing the viability of advanced flow batteries for grid-scale applications. For example, in 2019, the U.S. Department of Energy invested $24 million in a three-year program aimed at advancing flow battery technologies. One notable project within this program focused on using iron-salt electrolytes, a more cost-effective and abundant material compared to traditional options like vanadium. Preliminary results indicated a significant improvement in both efficiency and cost-effectiveness, signaling a positive trend for future deployments.
Commercial Implementations
Beyond pilot projects, some commercial implementations highlight the real-world feasibility of advanced flow batteries. One such instance is the RedT-avalon merger project in the UK, which aims to integrate solar power with vanadium flow batteries. The project successfully demonstrated that these systems could provide around-the-clock power to commercial establishments, thereby reducing dependency on the grid and fossil fuels.
Real-world case studies validate the theoretical advantages of advanced flow battery systems. These projects serve as tangible proof of concept and play a crucial role in attracting further investments and interest from both the public and private sectors. They lay the groundwork for scaling the technology and potentially revolutionizing how we think about energy storage for grid applications.
By examining these case studies, we get a glimpse into a future where flow batteries could be a cornerstone technology for sustainable energy systems.
Future Perspectives
Research Directions
While significant advancements have been made, there is still much to explore in the realm of flow batteries. Researchers are investigating various avenues to further optimize electrolyte chemistry, improve system architecture, and reduce manufacturing costs. Academic institutions and private labs are increasingly collaborating, ensuring a multi-disciplinary approach that addresses the technology from multiple angles.
Policy and Regulations
As flow batteries continue to prove their potential, there will be a growing need for policy frameworks that facilitate their widespread adoption. Incentives similar to those offered for solar power, such as grants or tax breaks, could be beneficial in encouraging utility companies and independent producers to invest in this technology. Regulatory standards around efficiency and environmental impact will also need to be developed to ensure safe and sustainable deployments.
The future of flow batteries looks promising, fueled by relentless research and innovation. As the technology matures, it stands to benefit not only from technological advancements but also from favorable policies and regulations that can expedite its commercial deployment. With their unique advantages and growing market potential, flow batteries could be at the forefront of the next big revolution in energy storage solutions, playing a pivotal role in our transition to a more sustainable and reliable energy ecosystem.
Conclusion
The journey towards sustainable and reliable energy storage solutions is fraught with challenges, yet it is crucial for the broader transition to renewable energy. Among the various technologies vying for prominence in this space, flow batteries have emerged as a compelling candidate, particularly for grid-scale applications.
While traditional flow battery systems have faced limitations in efficiency, cost, and scalability, recent advancements promise to overcome these hurdles. Innovations in material science are leading to more efficient and sustainable electrolytes. Concurrently, technological upgrades, particularly in control systems, are enhancing operational efficiency.
Case studies and pilot projects provide much-needed real-world validation, demonstrating the practical benefits and commercial viability of advanced flow batteries. As the technology continues to mature, it’s clear that both policy support and continued research will be instrumental in realizing its full potential.
Through comprehensive exploration—from the basic principles of flow batteries to their future outlook—we can appreciate the transformative potential of this technology. With ongoing advancements, flow batteries are poised to play an increasingly pivotal role in shaping a sustainable energy landscape, making them a technology well worth watching in the years to come.